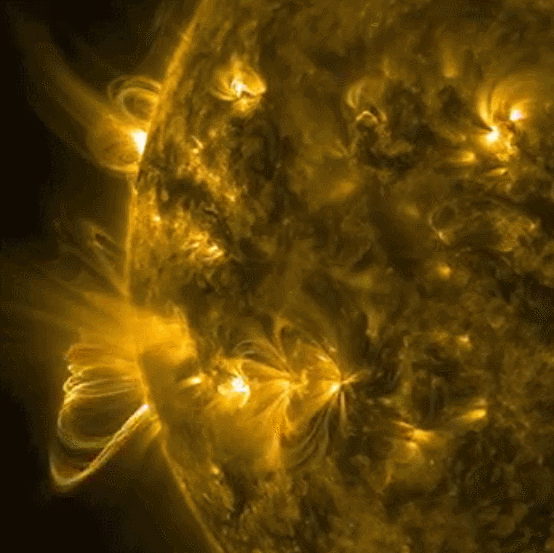
Imagine, you are sitting in front of a roaring campfire. You’ll feel the warmth of the flames. Now you back away from the inferno. You’ll feel cooler, right?
But this scenario doesn’t carry over in the case of the solar atmosphere. The surface of the Sun, which is called the photosphere, has a typical temperature of around 6000 Kelvin. Moving away from the surface of the Sun should cool the temperature down. But it has been observed that, at a height of around 2000 km above the surface, the corona, the temperature reaches a few million Kelvin, which is a thousand times higher than the temperature of the solar surface. This means that some mysterious processes are happening in the upper solar atmosphere that apparently can’t be explained by the (second) law of thermodynamics. This phenomenon is known as the “Coronal Heating Problem” of the Sun, which is one of the most challenging problems in solar physics. The story of finding such high temperatures in the solar corona is quite interesting. The corona can be observed from the Earth only during the time of the solar eclipse. During the solar eclipse in 1869, scientists observed a green spectral line emission from the corona, which was thought to be due to the presence of an unknown element. They thought that they have discovered a new element, and called it “Coronium” (From the name of Corona). For decades, this green line has been the da Vinci Code in the solar physics. After almost seven decades, it was confirmed that the cause for this green spectral line is due to the presence of iron, but in a 13 times ionized state (Fe +13 ). Scientists calculated that to be in such a high level of ionization, the temperature of the corona should be at least in the order of a million Kelvin. Since the discovery of the coronal heating problem, it has been realized that the puzzle is much more complicated than it appeared at first glance. This problem involves several complicated physical processes that are very difficult to observe or to capture in a theoretical model.
There are mainly two schools of thought to explain the coronal heating mechanism. The first group of scientists believes that the enormous amount of heat is created due to the dissipation of the magnetically driven waves in the higher atmosphere of the Sun. Let’s discuss this in more detail. The surface of the Sun, the photosphere, is consistently boiling and swirling with the mechanical energy through a process, called ‘convection’. As a result, the soup of hot charged particles, which is called the plasma, roil up through the solar surface — like the hot bubbles in a pot of boiling water. The motion of these moving plasma blobs creates a complex magnetic structure in the solar surface that extends up to the higher solar atmosphere. But here the question arises is: why the magnetic field lines care for the plasma? the answer is the following: due to the presence of large length scales and high electrical conductivity, the plasma and the magnetic fields are glued together in the solar atmosphere (unlike the case of the laboratory plasma). Which means, where ever the plasma medium moves, the magnetic field lines are dragged with it — like the Romeo-Juliet combo; they can’t get separate. This phenomenon is a consequence of a magnetic property in the solar atmosphere, called “Flux Freezing”. Well, this scenario reminds me of an old TV commercial of Hutch (a telecommunication company), where a puppy used to follow his master with the tagline: “where ever you go, our network follows”. In the case of flux freezing: if the puppy is thought to be the magnetic field lines, then the plasma medium may be considered as the master of the puppy. The theory proposes that the convective motion of the plasma produces a magnetic wave of a certain frequency — called Alfvén waves — that propagates through a magnetic channel of a cylinder-like structure — called the “magnetic flux tube”, and dissipates in the higher atmosphere in the form of kinetic and thermal energy. On the other hand, the second group of scientists believe that the main reason behind the coronal heating is bomb-like explosions — called “solar flares”. Due to the turbulent boiling of the plasma blobs, the magnetic footpoints in the solar surface carry out random motions — like the hot water bubbles move in a pan of boiling water. And as the field lines are stuck with the plasma material, the magnetic field lines and its larger counterparts — the magnetic flux tubes, twist and wrap among themselves developing the magnetic stress and tension — like two rubber bands wrapping among themselves acquire a potential energy. When the wrapping reaches a certain limit and the magnetic stress crosses a threshold, a part of the field lines or the flux tubes breaks out and snap explosively in the form of energy — like breaking of the rubber band. So we see that the study of the magnetic flux tubes is one of the key aspects for revealing the coronal heating problem that I have addressed in my research. Magnetic flux tubes are basically a cylindrical-like structure, enclosed by a simple surface that contains magnetic fields (see Figure 1). Magnetic flux tubes should not be thought of as isolated structures. A magnetic region is formed by an ensemble of the flux tubes.

Figure 1: A cartoon diagram of a magnetic flux tube. The number of field lines entering the surface S1 and crossing S2 are same (Figure source: Wikipedia).
An important aspect of the theoretical modeling of a magnetic flux tube is its stability of equilibrium. Flux tubes need to be in an equilibrium state in the solar atmosphere. This phenomenon can be related by an analogy of a balloon full of kerosene dumped inside a water vessel. If the balloon doesn’t burst, then we can say that the balloon is in equilibrium with the water outside. Now, if we start freezing the water of the vessel, after a while we will see that, the balloon bursts. This is because, when the water starts to be freeze, it exerts more pressure in the balloon, and after a pressure threshold, the balloon can’t withstand the pressure difference between the internal and external fluids and bursts. A quite similar scenario happens in the case of magnetic flux tubes. The total pressure inside the flux tube, which is due to the contribution of plasma and magnetic field, matches with the pressure outside the flux tube medium; which keeps the flux tube in equilibrium. Now, if the internal or the external pressure change, the flux tube tries to achieve a new equilibrium state unless the pressure difference exceeds the threshold and breaks out. In our research, we have developed a new magnetohydrostatic equilibrium solution of flux tubes by incorporating the appropriate boundary conditions, which are relevant to the solar atmosphere. We have also calculated the magnetic and thermodynamic structures of the flux tube and shown that the results are in reasonable agreement with the observations of the solar atmosphere. The model we have developed is a generic flux tube model that can be branch out into two distinct magnetic structures — which we call the open field and closed field flux tubes. If the magnetic field lines rise but do not return to that same plane within the simulation domain, then the field lines look like an open structure. On the other hand, the field lines that rise and fall back in the same horizontal plane, form a closed magnetic structure (see Figure 2). Both the open and closed configuration flux tube models have important aspects in the solar physics. The magnetic waves that dissipate in the higher atmosphere, propagate through the open field flux tubes. Whereas the closed field flux tube model can be used to construct realistic structures like Coronal loops or magnetic canopies. Figure 3 shows an image of coronal loops, where the field lines are bipolar and form a loop-like structure that spans from the photosphere to the corona. The flux tube models that we have developed are the recipes that can be directly fed into the computer simulation to study the wave dynamics for calculating the amount of energy budget it is dumping in the solar atmosphere or several other important properties of the Sun.

Figure 2: Theoretical predictions of the 3D configuration of the open (left column), and closed (right column) field lines inside the magnetic flux tubes obtained by our model.
The coronal heating problem shouldn’t be studied only for the astrophysical interest. Scientists have proposed that the astrophysical plasma (which has a very high Reynolds number) can also be produced in the laboratory, though the processes are extremely challenging. Therefore, if the exact mechanism of the coronal heating problem is understood, then the same mechanism can be replicated in the terrestrial laboratory to generate a huge amount of energy, which might solve the energy deficiency problem of the Earth. Though it’s hard to predict, how the solving of the coronal heating mystery will change our understanding about the space around us, but the fundamental discoveries like this will certainly uplift the gear of science and technology to a new level.

Figure 3: Coronal loops: an example of flux tubes observed in the solar atmosphere (Image courtesy: Vatican Observatory Foundation).